Time Division Multiplexing
(TDM) versus Wavelength Division Multiplexing (WDM)
Ultrahigh-speed photonic
networks capable of accommodating the increase in Internet data
traffic will form the infrastructure of the information society
of the next generation. There are two types of multiplexing schemes
to accommodate such large amount of information: wavelength division
multiplexing (WDM), which multiplexes signals using lightwaves
with different wavelengths, and time division multiplexing (TDM),
which multiplexes signals in different bit slots in the time domain.
In WDM systems, transmitters and receivers in each channel work
independently, and thus WDM allows signals with different format
to be accommodated in one network. In this sense, WDM is an "analog"
multiplexing scheme. In constrast, TDM requires sophisticated
signal processing employing, for example, multiplexers, demultiplexers,
clock recovery, and network synchronization. Nevertheless it supports
"digital" multiplexing, where synchronized high speed
signals are processed together. Optical TDM (OTDM) makes the most
of these advantages in the optical domain and is another important
technique for the construction of photonic networks in addition
to the development of highspeed signal processing.
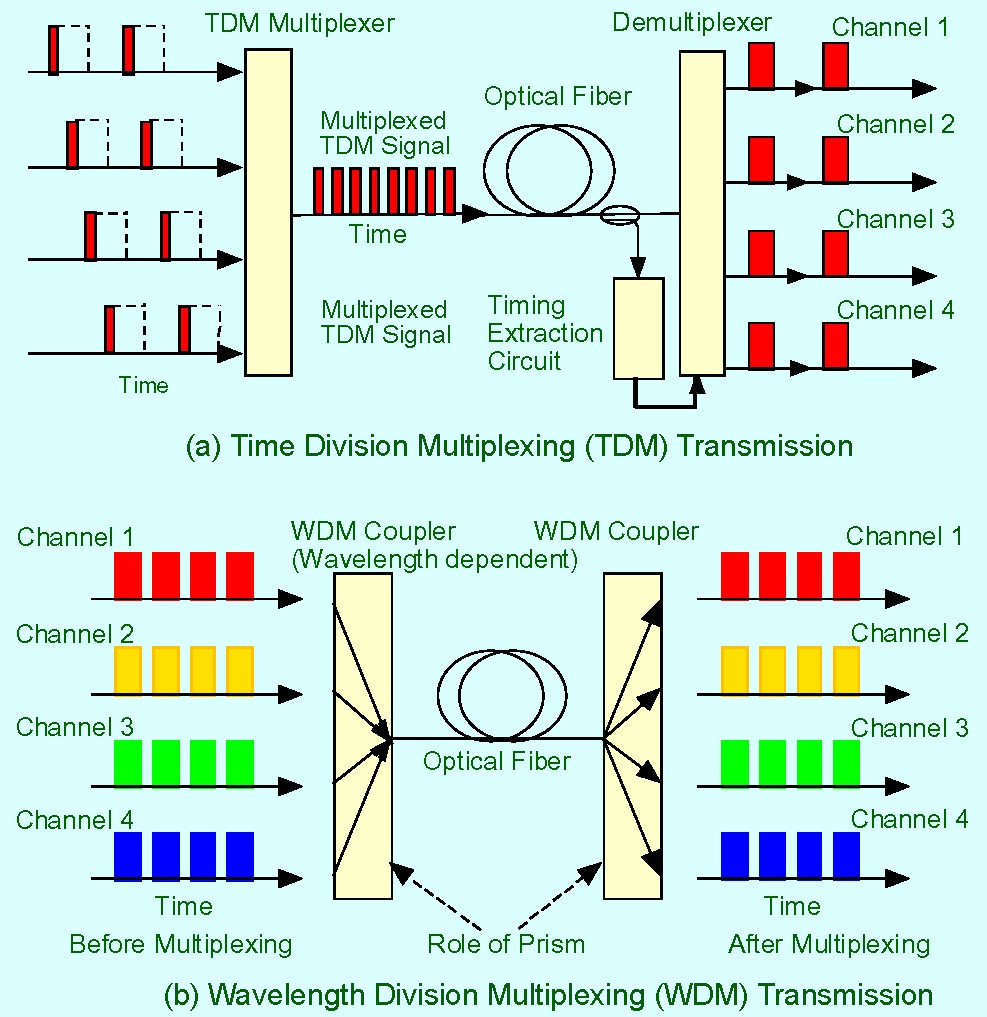
Fig. 1 TDM versus WDM
1.28 Tbit/s OTDM signal transmission
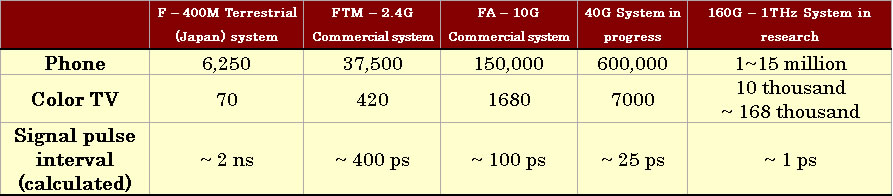
Fig. 2 Optical transmission systems and signal pulse interval.
Figure 2 shows the improvement
in the TDM transmission speed in backbone terrestial optical transmission
systems in Japan. The transmission speed has increased from 400
Mbit/s to 2.4 Gbit/s and 10 Gbit/s. With the help of WDM, the
capacity can be increased further. Work on a 40 Gbit/s system
is currently in progress and it will be installed in the backbone
system in the near future. This system benefits from the development
of high speed electronic devices.
The next research target is ultrahigh-speed
OTDM transmission with a bit rate of 160 Gbit/s or even 1 Tbit/s,
where highspeed signals are multiplexed in the optical domain
alone, without the need for any electronic devices. OTDM transmission
operates in a regime far beyond the capability of electronic devices.
In this regime ultra short pulses are transmitted with pulse widths
of pico second to a few hundred femto second order. This would
be impossible without the development of advanced technologies
such as the generation of femto-second pulses, higher-order dispersion
compensation, and all-optical demultiplexers.
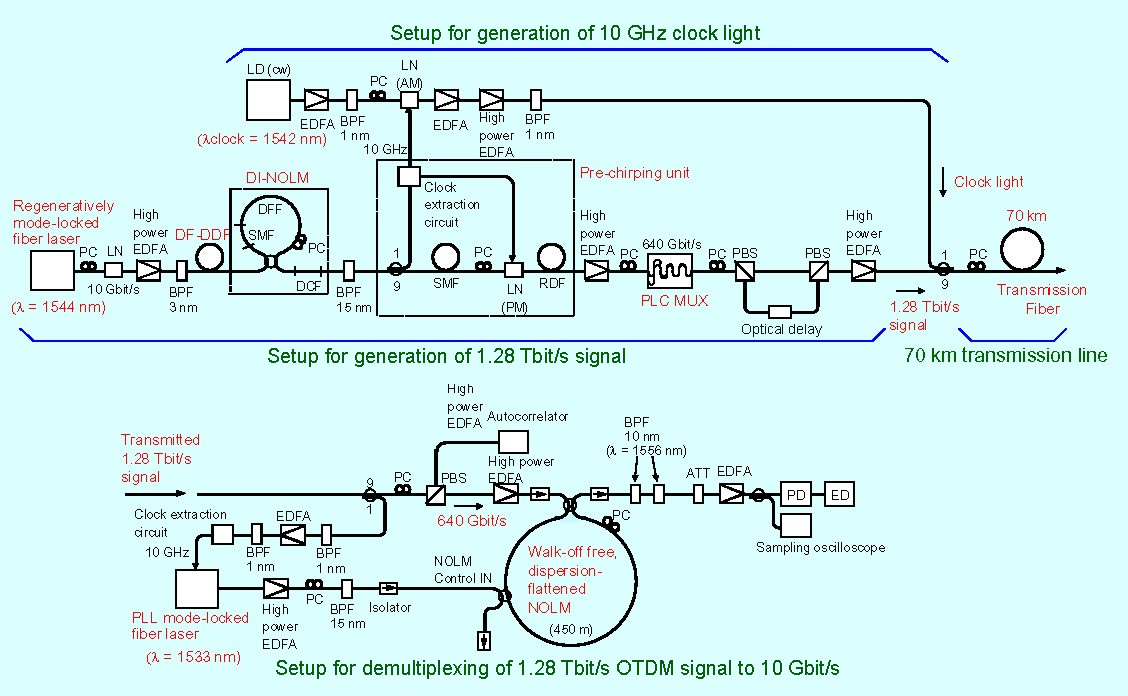
Fig. 3 Experimental setup for 1.28 Tbit/s OTDM signal
transmission.
Figure 3 shows our setup
for a 1.28 Tbit/s-70 km OTDM transmission experiment, which was
successfully achieved for the first time in the world. A 3 ps,
10 GHz regeneratively and harmonically mode-locked fiber laser
operating at 1.544 mm was used as the original pulse source. The
output laser pulse was intensity-modulated at 10 Gbit/s and the
pulse train was coupled into a dispersion-flattened dispersion
decreasing fiber. This realized adiabatic soliton compression
to less than 200 fs. We incorporated a phase modulation technique
that compensated for the third- and fourth-order dispersion of
the transmission fiber. The pre-chirped 10 GHz pulse train was
optically multiplexed to 640 Gbit/s by using a planar lightwave
circuit (PLC). We obtained a 1.28 Tbit/s signal by polarization
multiplexing two 640 Gbit/s pulse trains.
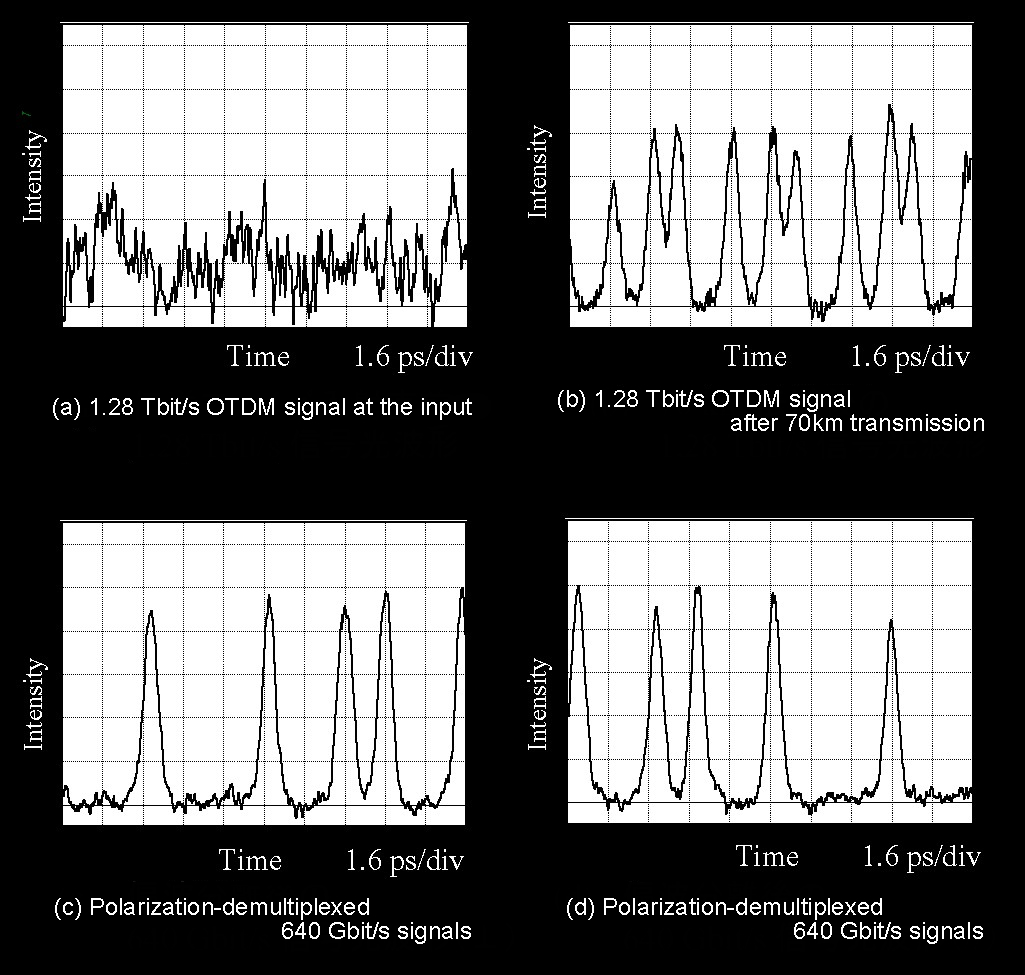
Fig. 4 Optical signal waveform in 1.28 Tbit/s OTDM signal
transmission.
Fugure 4 shows the input and output data patterns. Clean 640
Gbit/s signals were obtained in each channel. The pulse broadening
after 70 km transmission was only 20 fs. We obtained a bit
error rate of 10-9 was achieved for all the channels.
|